MRFM
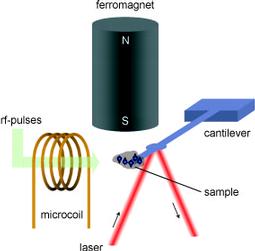
Magnetic resonance force microscopy (MRFM) is a method to image the spin density, pioneered by John Sidles and Dan Rugar. MRFM is similar to magnetic resonance imaging (MRI), but it is adapted for imaging in the micro- and nanometer range. In fact, whereas MRI uses inductive detection and is presently limited to the detection of volumes larger than 1 μm by sensitivity, MRFM is based on mechanical detection of the magnetic forces produced by nuclear moments. Since very small forces can be measured, MRFM can in principle detect at resolutions far below 1 μm.
MRFM relies on the mechanical measurement of the force arising between the nuclear (or electron) magnetic moment of a sample placed in a static magnetic field, and the strong magnetic field gradient produced at the sample position by a small ferromagnet. This small force acts on the cantilever, a small flexible beam of silicon, and it can be inverted with the rf-pulses, flipping the magnetization of the spins in a selected spatial region by 180o. These inversions are performed periodically at the eigenfrequency of the cantilever, exciting it at resonance. The displacement of the cantilever is detected by a laser beam deflected off its surface.
Our main emphasis is on exploring the possibilities of combining spectroscopic concepts with MRFM imaging. In fact, MRFM is not limited to the three spatial dimensions and spectroscopic dimensions can be added, providing detailed chemical and structural information at the atomic level. For example, spectral information based on homo- and heteronuclear dipolar interactions as well as on the chemical shift can be used for contrast generation. In order to exploit this "chemical shift information", however, high spectral resolution is needed. We have then developed a probe where the gradient source can be temporarily moved away during the experiment, so that he spectroscopic information can then be collected in a nearly homogeneous field. We demonstrated chemical-shift imaging of solids with a spatial resolution of about 1 µm using MRFM and we recorded images with two spatial dimensions and one spectral dimension and with three spatial dimensions.
Our current projects are aimed at developing the MRFM technology and enabling applications in research fields like biology and material sciences.
- Imaging polymers blends. The study of the 3D structure of polymer blends is challenging since sub-micrometer resolution is typically needed. Compared to other imaging techniques, MRFM has the advantage of being non-invasive and not limited to the surface of the sample, and that contrast can be generated by spectroscopic techniques. On the other side, the current implementation of our apparatus uses spin-lock to modulate the Zeeman magnetization and, thus, T1-rho is a limiting factor. We developed significant improvements to the previous MRFM excitation schemes and we applied these schemes to a polymer blend of 80% PEEK and 20% PTFE acquiring full-volume 2D spatial images (with Hadamard multiplexing) of both the 1H and 19F-rich phases with a 6-fold enhancement in the signal-to-noise ratio.
- Measuring samples containing water, e.g. biological cells. Our setup is designed to work at room temperature, thus allowing, in principle, to investigate systems which contain water, such as crystalline proteins (which contain about 50% water) and biological cells. For these experiments it is necessary to isolate the sample from the high vacuum condition necessary at the location of the cantilever. Such a setup is possible as the MRFM microscope can easily “see” through a ceramic window of a few 100 nm thickness and necessitates a gradient-on-cantilever geometry. Once established an appropriate protection system, in-vivo imaging of large cells and the measurement of local water diffusion coefficients should be possible with the resolution of an optical microscope and with the availability of several spectroscopic contrast possibilities like the ones based on T1.
- Methods development: 2D, 3D with coils as gradient sources for the second and third dimension and more efficient read-out schemes (quadrature detection, inversion sweeps).